On Earth a variety of tiny lifeforms float and drift in the air at the mercy of the winds, just like marine plankton that are at the mercy of the currents. However, what goes up must come down and they ultimately return to the ground. If an aerial ecosystem involving lighter-than-air filter feeding gasbags is to exist then aerial plankton should be much richer than on Earth and likely adapted to perpetual living in the clouds. This article fits within a wider series on lighter-than-air-life.
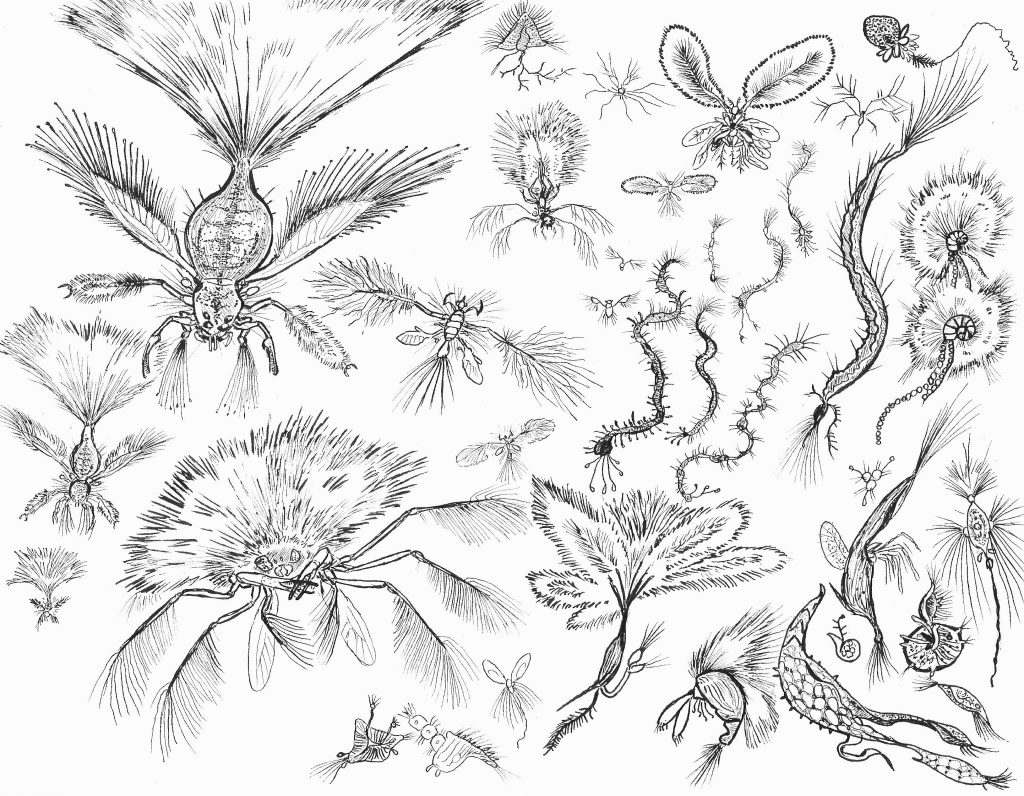
Gasbag Series Articles
- Part 1: Physics
- Part 2: Aeroplankton
Summary
This article follows on from the previous article on lighter-than-air life (aka gasbags). However, that article focused on larger and/or multicellular type life. In contrast, this article will consider smaller and/or unicellular life. It is worth at least glancing at the other article first to get a background on the general concepts of buoyancy if you are not already familiar with them.
The biodiversity and biogeography of the atmosphere on Earth have been relatively under investigated, however, it does seem plausible that skies on a fictional world could be filled with planktonic life just like the oceans.
Noi viviamo sommersi nel fondo d’un pelago d’aria. We live submerged at the bottom of an ocean of air.
A brief investigation of several concepts leads to the following key points that may help guide any speculative evolution or world building that requires floating life in the clouds. After all, without small floating plankton what would the larger gasbags eat?
- Aerial plankton can be split into floaters and sinkers
- Single celled life could live in water droplets as they rise and fall through the atmosphere
- Small (> 10 μm) lighter-than-air life is only feasible using graphene
- Hydrogen filled single cells could float when they are larger than raindrops (> 2 mm)
- Air filled single cells up to 100 μm can remain aloft with updrafts of 1 cm/s
- Larger, hairy, multicellular life can soar on updrafts if they are ten times faster
- Electrostatic repulsion can be used to make life of various sizes float
- Conditions on other planets could making aerial living more viable
Background
The skies of Earth are filled with various lifeforms that have no control over their direction and can only drift with the wind. These are collectively known as aeroplankton and consist of a wide range of seeds, pollen, spores, fungi, protists, bacteria and even viruses. These can be blown into the air via marine sea spray or terrestrial dust storms.
However, while they may stay in the air for a few days, eventually they will all come down. No life on Earth is believed to permanently live in the clouds but would it be possible for life to adapt to perpetually soar the skies without powered flight? A key desire for such aeroplankton is that the gasbags described in the previous article are often envisaged to be filter feeders. Therefore a diverse and bountiful first step in a food web would certainly help to support them.
Even back in 1913, Sir Arthur Conan Doyle recognised the need for something similar to justify the large airborne creatures encountered by Joyce-Armstrong, the aviator hero of the The Horror of the Heights*:
… The air in front of me had lost its crystal clearness. It was full of long, ragged wisps of something which I can only compare to very fine cigarette smoke. It hung about in wreaths and coils, turning and twisting slowly in the sunlight. As the monoplane shot through it, I was aware of a faint taste of oil upon my lips, and there was a greasy scum upon the woodwork of the machine. Some infinitely fine organic matter appeared to be suspended in the atmosphere. There was no life there. It was inchoate and diffuse, extending for many square acres and then fringing off into the void. No, it was not life. But might it not be the remains of life? Above all, might it not be the food of life, of monstrous life, even as the humble grease of the ocean is the food for the mighty whale?”
This article intends to investigate the possibility of perpetual aeroplankton and how they might remain airborne for their entire lifecycle.
Hitchhikers
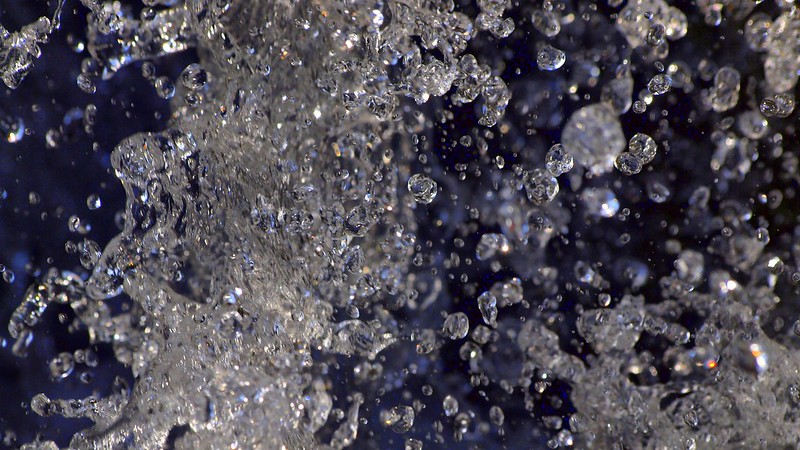
The first strategy for producing perpetual life in the clouds is similar to what some Earth aeroplankton already do. That is they hitch a ride in water droplets which are carried upwards with convection before falling as rain once they become too large. This approach has recently been comprehensively described for potential life in the Venusian lower atmosphere haze.
The proposed mechanism on Venus would work as follows:
- Desiccated spores populate the lower atmosphere haze layer.
- Spores in the lower haze layer are carried to the lower clouds by vertical wind motion
- The spores act as cloud condensation nuclei and once surrounded by a liquid droplet germinate to a metabolically active life-form
- This life-form lives in the droplet for months to years and during this time the droplet grows by coagulation
- Once the droplet is too large to remain aloft, it settles down to lower layers, where heat evaporates the water triggering cell division and sporulation
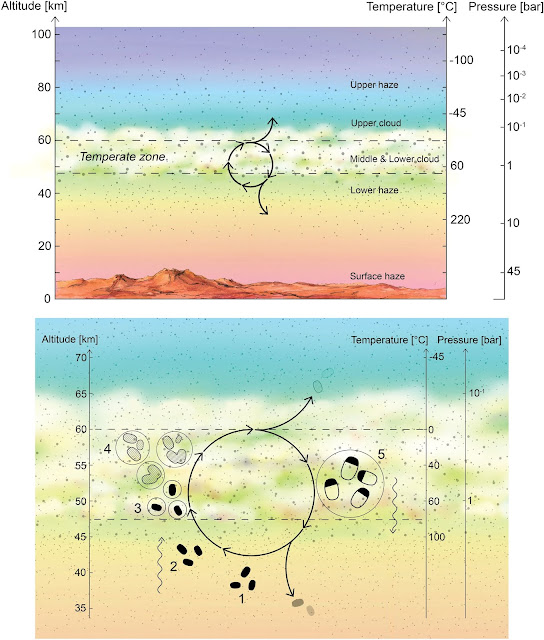
This process is slightly different to the one that occurs on Earth as the water droplets wouldn’t evaporate before they hit the ground. The general principle remains the same as instead of the lower haze layer acting as a holding zone for spores, the ground or sea could instead act as a launch pad for spores beginning their upward journey. Since life already does this it requires no further justification to include it in any speculative world building.
On worlds with permanent clouds (i.e. Jupiter, Venus and Titan like worlds) it becomes even easier to justify this as a permanent phenomenon. In contrast on worlds like Earth the biomass contained in the clouds would regularly decrease through rain which would need to be replaced with new life launched from the ground.
This could mean that filter feeding gas bags would preferentially graze clouds full of large droplets before they can fall as rain as this would be where the richest feeding could be found. It’s therefore perhaps not implausible that their actions would have an effect on the meteorology of the planet. They could consume large droplets before they fall and produce smaller ones behind them thus preventing rain and encouraging the cycle to begin anew.
This approach is suitable for most planets though, depending on atmospheric conditions, it may require a portion of the lifestyle to be spent on the ground therefore may not technically be perpetual.
Floaters and Sinkers
Instead of hitching a ride it may also be possible for life to exist “naked” in the clouds. Admittedly, there is always the possibility that it would act as a condensation nucleus for a water droplet and would end up hitching a ride anyway. In order to consider whether potential aeroplankton will float or sink there are four forces that should be considered:
- Buoyancy
- Gravity
- Drag
- Electrostatic
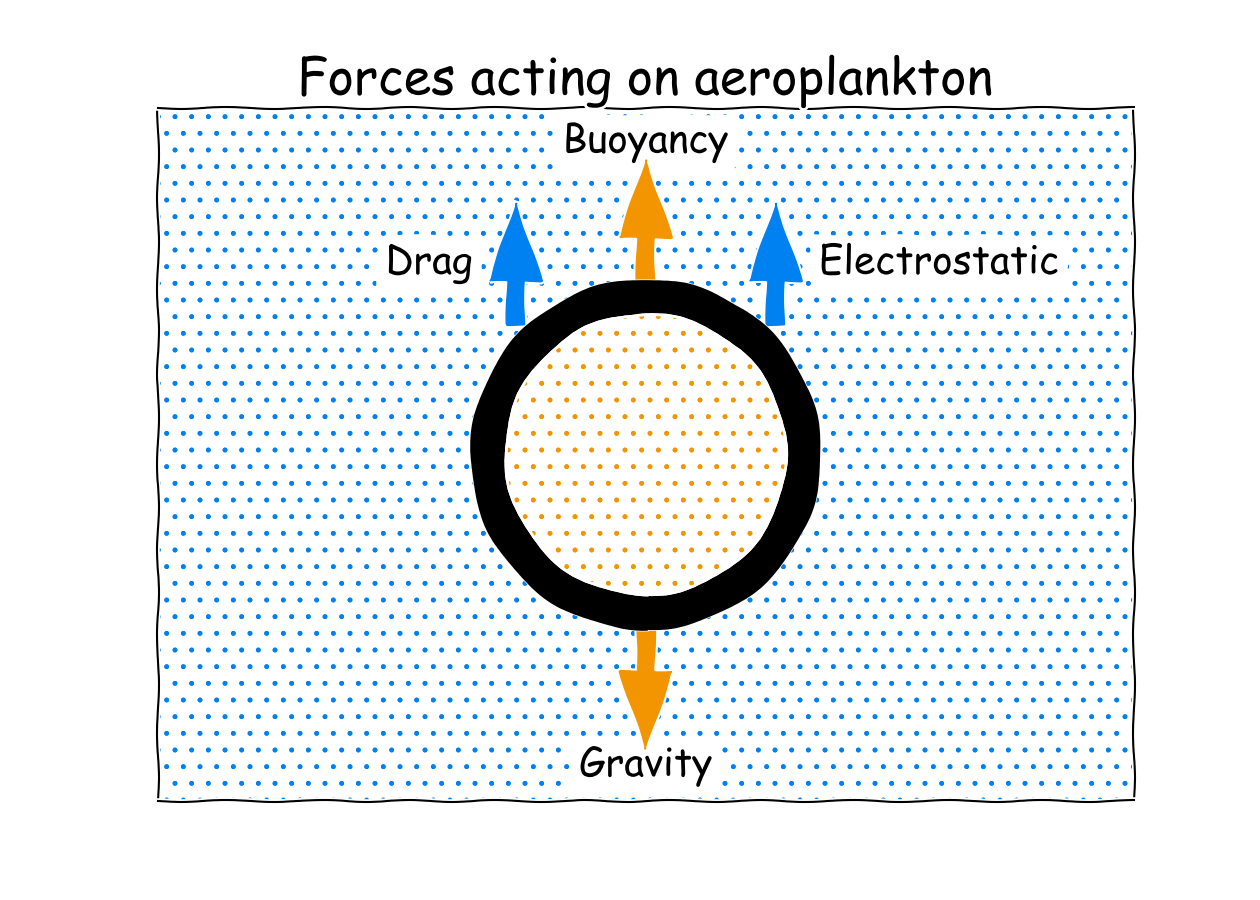
Buoyancy was covered in the previous article on larger gasbags and it refers to lighter-than-air organisms floating upwards. In contrast, gravity is the force that will pull aeroplankton downwards. Drag could operate in either direction as it is the tendency of the atmosphere to resist motion in any direction. However, here it will typically be a force acting upwards to partially oppose gravity making aeroplankton sink to the ground. Finally, an unexpected factor exists when the aeroplankon is electrically charged in opposition to the ground as this can push it upwards, against gravity.
Each of these forces will be considered in turn though in reality they will all simultaneously apply to any organism in the air. Drag is perhaps the most awkward one though as fluid mechanics can be quite complicated. In particular, fluids (i.e. air) can behave differently when the length scale and velocities of interest change. This concept is embodied in the Reynolds number and is particularly important when considering small aeroplankton.
The chart below shows lines of equal Reynolds number for the speeds and length scales of interest. For slowly falling tiny aeroplankton the Reynolds number is much less than one so the drag force equation dominated by viscous forces applies. In contrast, for larger aeroplankton the Reynolds number is larger and so the drag equation dominated by inertial forces may apply instead.
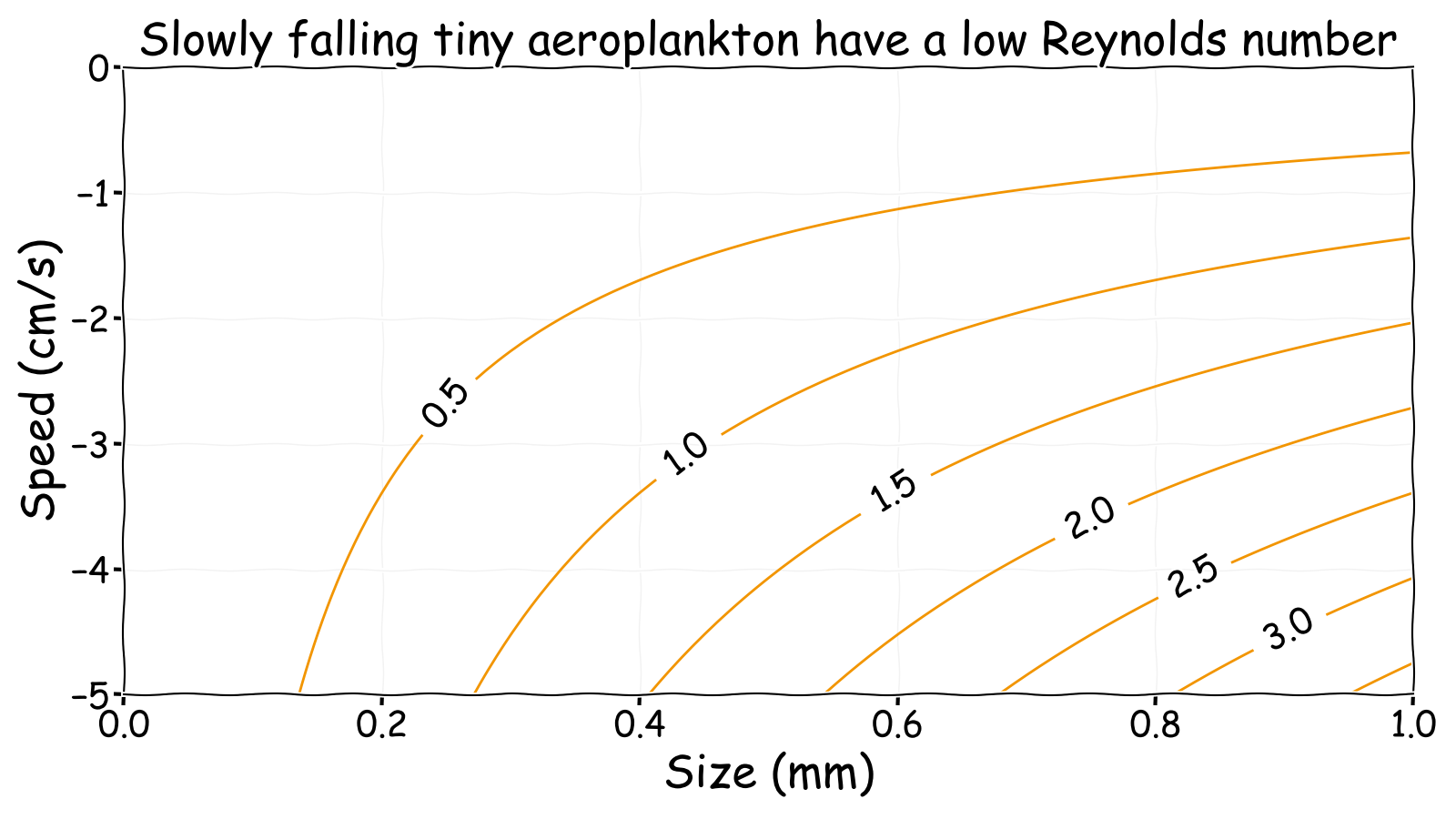
Aeroplankton Concepts
As an illustration, six simple aeroplankton concepts are presented. For each of them a single diameter value determines their size. This value is expected to vary between 1.0 μm to 100 μm. This is equivalent to the range of typical small to large aerosol particles with cloud droplets being around 20 μm and raindrops about 2 mm across.
The first type of a cell is just a single solid cell with a variable diameter. Clearly this will never be neutrally buoyant but it is included for comparison later.
- Small sphere: Single spherical cell of variable diameter
The second type of cell is a small gasbag with a hydrogen filled “magic material” graphene balloon which carrying a 1.0 μm diameter cell underneath.
- Aerographene bubble: Hydrogen filled aerographene bubble with a cell underneath
The third type of aeroplankton are hollow cells that contain a gas vacuole surrounded by 0.5 μm of cytoplasm.
- Air vacuole: Air filled vacuole
- Hydrogen vacuole: Hydrogen filled vacuole
A modified version of this is inspired by diatoms which are surrounded by silica frustules. Since leaking hydrogen may be a problem this might reduce the leakage rate slightly as some silica glasses have lower permeability to hydrogen. In addition, silica can potentially hold an electric charge to take advantage of electrostatic lift.
- Silica vacuole: Hydrogen filled vacuole and a 0.5 μm thick silica cell wall
The final aeroplankton concept is a larger multicellular organism to which a large Reynolds number applies. For simplicity this will be assumed to be a disc shape.
- Large disc: Multicellular organism spread out into a flat disc-like shape
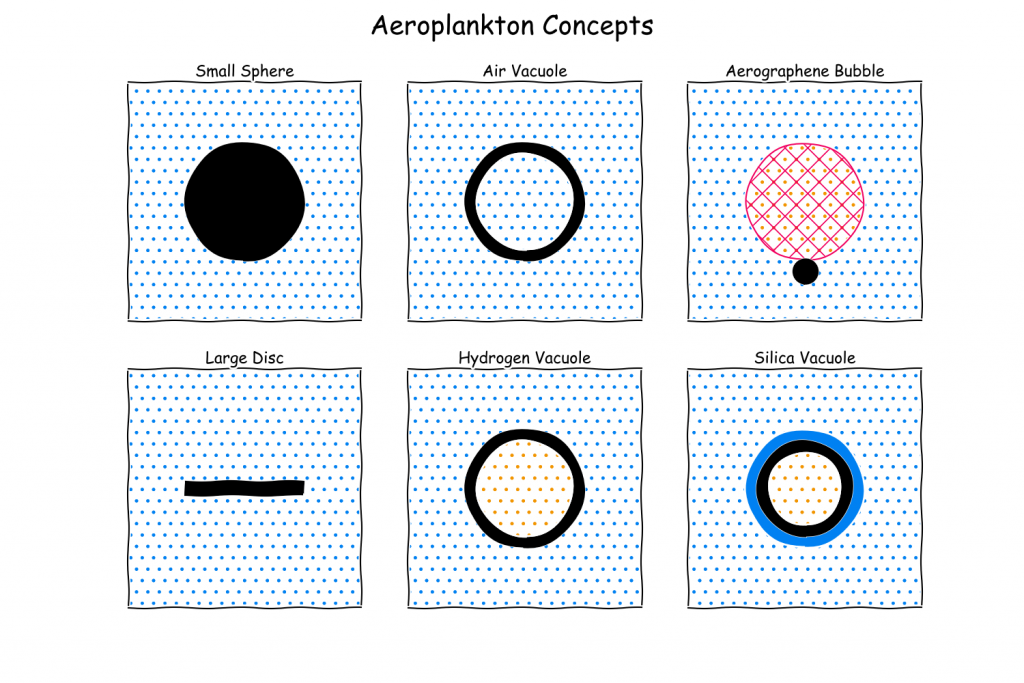
While a 1.0 μm diameter cell or a 0.5 μm thickness shell around a vacuole might seem small the tiny prochlorococcus cyanobacteria is only 0.6 μm in diameter and yet is probably the most abundant photosynthetic organism on Earth. Therefore these concepts seem somewhat viable as a lower limit on size.
Buoyancy
Since the cell cytoplasm is basically water and water is denser than air then the only concepts that might be neutrally buoyant are the three that contain hydrogen. The buoyancy of each of these designs can be calculated using the same approach as previously for larger gasbags. If the weight of the organism (including any gas present) is less than the weight of the air displaced then the aeroplankton will be lighter-than-air and will float upwards until it reaches a region with lower air density and becomes neutrally buoyant.
\[\operatorname{apparent\_mass} = \operatorname{organism\_mass} – \operatorname{displaced\_air\_mass}\]
As expected, only the amazing properties of graphene allow lighter-than-air organisms at plankton-like sizes. Aerographene bubbles over 10 μm in diameter become lighter-than-air. Effectively the aeroplankton has created floating fine silt!
In contrast the hydrogen filled vacuole concept has to be the size of a raindrop with the silica coated version even larger. While this may sound a bit big it is worth comparing it to bubble algae (Valonia ventricosa). This single celled algae is similar in structure as the vacuole concept except it is filled with water and lives under the sea. They can grow to be a few centimetres across and the peripheral cytoplasma is only 0.04 μm thick rather than 0.5 μm.
Hydrogen filled aeroplankton floaters may well be possible as either dust-like graphene motes or larger spheres visible to the naked eye.
Drag (Small)
While lighter-than-air floaters are somewhat plausible it seems more likely that the majority of aeroplankton would adopt the “sinker” strategy used on Earth. This involves the aeroplankton being heavier-than-air and slowly sinking through the atmosphere.
By increasing the atmospheric drag they can reduce the sinking rate to a low level so that vertical wind can push them upwards. This is after all the reason that water droplets on Earth don’t immediately fall out of the sky since they are obviously heavier than air.
The downwards force from gravity can be calculated from the effective weight of the organism
\[F_{gravity} = -(M – V\rho_{air})g\]
\(M\) is the total mass of the organism (including the lifting gas), \(V\) is the total volume (including the bubble) and \(\rho_{air}\) is the density of the surrounding air.
For small aeroplankton (i.e. low Reynolds number) the upwards force caused by drag is defined by Stokes’ Law which describes the viscous drag experienced by small smooth spheres moving through a fluid. Real aeroplankton will probably have rough surfaces to further increase drag though.
\[F_{drag} = 3\pi \mu Dv\]
\(\mu\) is the viscosity of the air, \(D\) is the diameter of the spherical organism and \(v\) is the velocity of motion through the fluid.
This drag force opposes the (downward) motion of the aeroplankton that is caused by gravity. Since it is proportional to velocity as gravity makes the aeroplankton fall faster and faster the drag force will become stronger and stronger. Eventually a point will be reached where the two forces are equal. The velocity at this point is the terminal velocity and the particle will fall no faster.
\[v = -\frac{(M – V\rho_{air})g}{3\pi \mu D}\]
This terminal velocity is relative to the speed of the fluid around the aeroplankton. Therefore, if the downward terminal velocity is equal to the upwards wind speed then the aeroplankton will remain at a fixed altitude. Alternatively, if the terminal velocity is only slightly faster than the upward wind speed then the aeroplankton will fall very slowly. It may never reach the ground before it divides into two smaller cells which will then drift upwards again.
The terminal velocity can be minimised if the aeroplankton can become larger without become more massive. This is achieved via a vacuole and the following chart shows the terminal velocities for the three vacuole concepts.
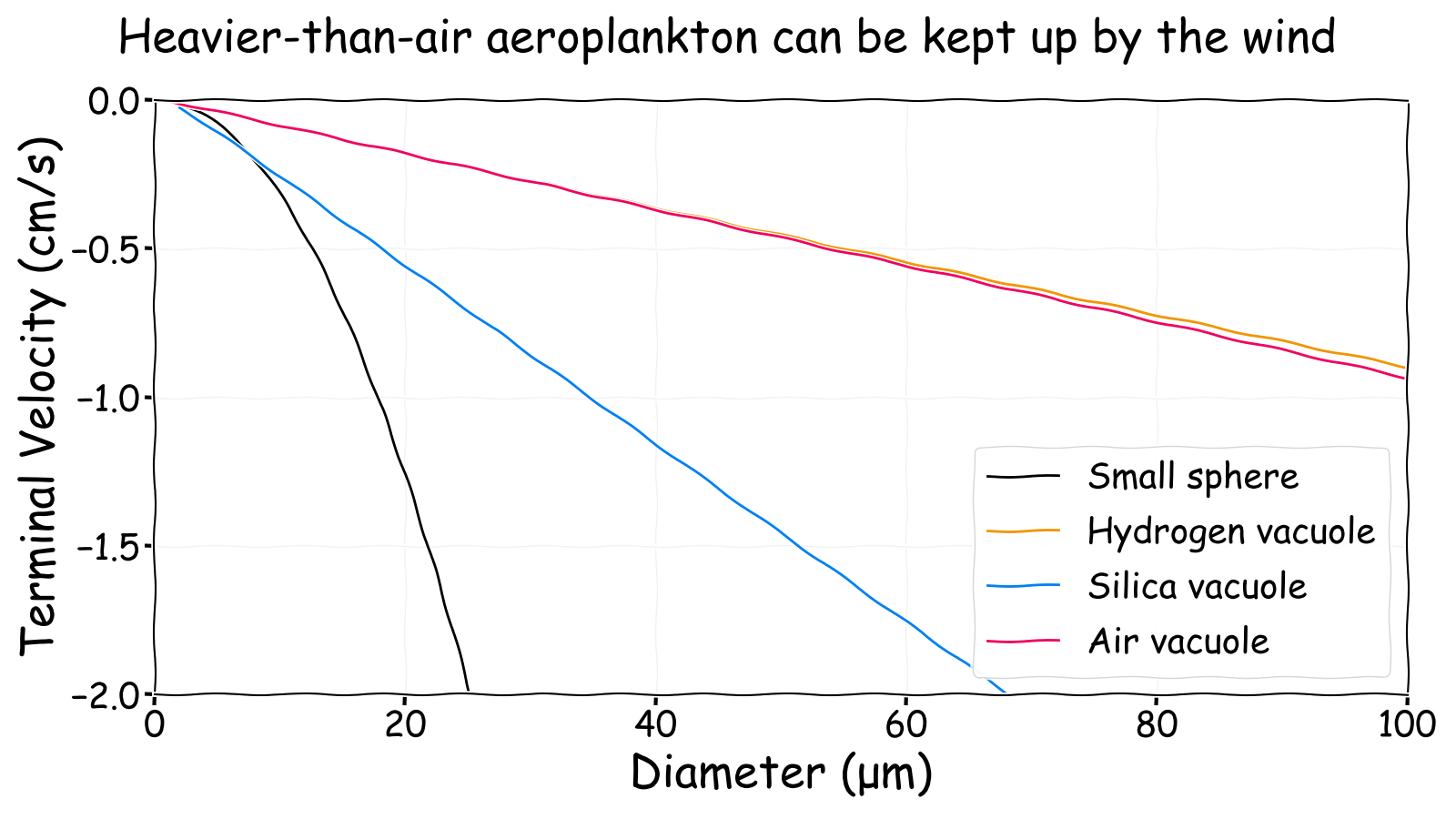
I can’t find a consistent value for typical upward wind velocity but quoted values of a few centimetres per second seem reasonable though in windy or stormy conditions this would be much higher. It turns out that any of these concepts within the expected size range could remain airborne with vertical winds of these speeds. The denser concepts such as the solid cell and the silica coated vacuole would have to be smaller whereas the air or hydrogen filled concepts can be larger. Interestingly the use of a hydrogen vacuole barely reduces the terminal velocity relative to an air filled vacuole across these sizes.
Changing the atmospheric conditions to those found on another planet changes things slightly. As before with the lighter-than-air gasbag analysis, Titan provides more lift than on Earth and Jupiter provides less. However, there are also small changes in viscosity which will effect the sinkers too. It is slightly more complicated to calculate the viscosity for exoplanet atmospheres, so I won’t do that now. The following guidelines may help though:
- Pressure: Viscosity changes little with pressure near Earth normal.
- Temperature: Unlike liquids, gas viscosity increases with temperature.
- Composition: Oxygen and nitrogen are the most viscous common atmospheric gases, whereas hydrogen is the least viscous.
- Humidity: Viscosity decreases with increased humidity.
Interestingly, this means that on a high gravity planet with a thick atmosphere small sinkers would have to be lighter than they are on Earth. This is because gravity pulling them down is stronger but the drag keeping them up remains the same as viscosity changes little with pressure.
This suggests that small sinker aeroplankton containing a vacuole would be viable on any planet though the size would vary slightly.
Drag (Large)
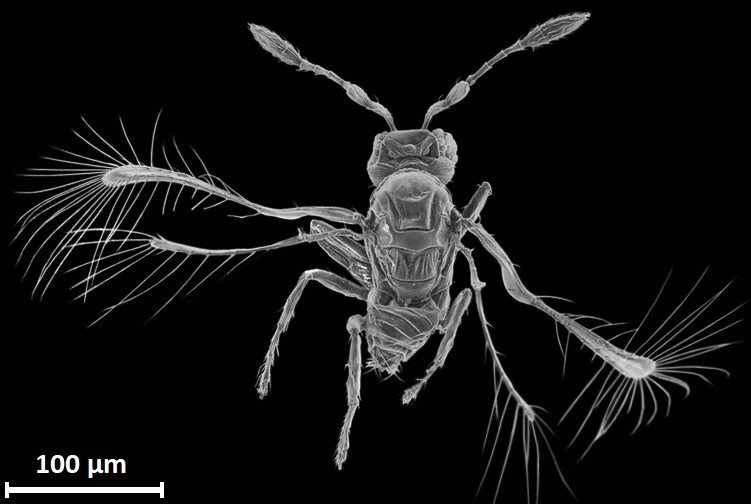
In the previous section, the solid cell and air vacuole concepts will never be lighter-than-air and so must always rely on vertical air motion to remain in the air. For small cells on the scale of water droplets this is viable, however, can larger organisms do this too?
The Kikiki huna fairyfly is the smallest flying insect at a length of 150 μm (with other fairyflies up to 1 mm in size) but note how its wings look like hairy paddles. As the opening artwork by 123Thundernugget also suggests, making the body more hairy may increase drag sufficiently to remain in the air without necessarily needing to flap wings (though that could also be done).
With the aid of hair to increase their effective cross section without significantly increasing their mass, organisms could increase drag without also increasing the downward gravitational force. As before, the end result of this is a lower terminal velocity. Since the Reynolds number is larger for these larger organisms the terminal velocity is approximately defined by the more traditional term (without including buoyancy).
\[V_t = \sqrt{\frac{2Mg}{\rho_{air}AC_d}}\]
\(M\) is the mass of the organism, \(g\) is the acceleration due to gravity, \(\rho_{air}\) is the density of the air, \(A\) is the projected area of the organism and \(C_d\) is the drag coefficient. The drag coefficient is the interesting parameter as it depends on shape. Assuming the organisms are thin discs (and not spherical cows) with a ratio of diameter to thickness of 100 gives a high drag coefficient of 1.12. In practice since the Reynolds number is still quite low the drag coefficient should be larger but this will do for a crude estimate.
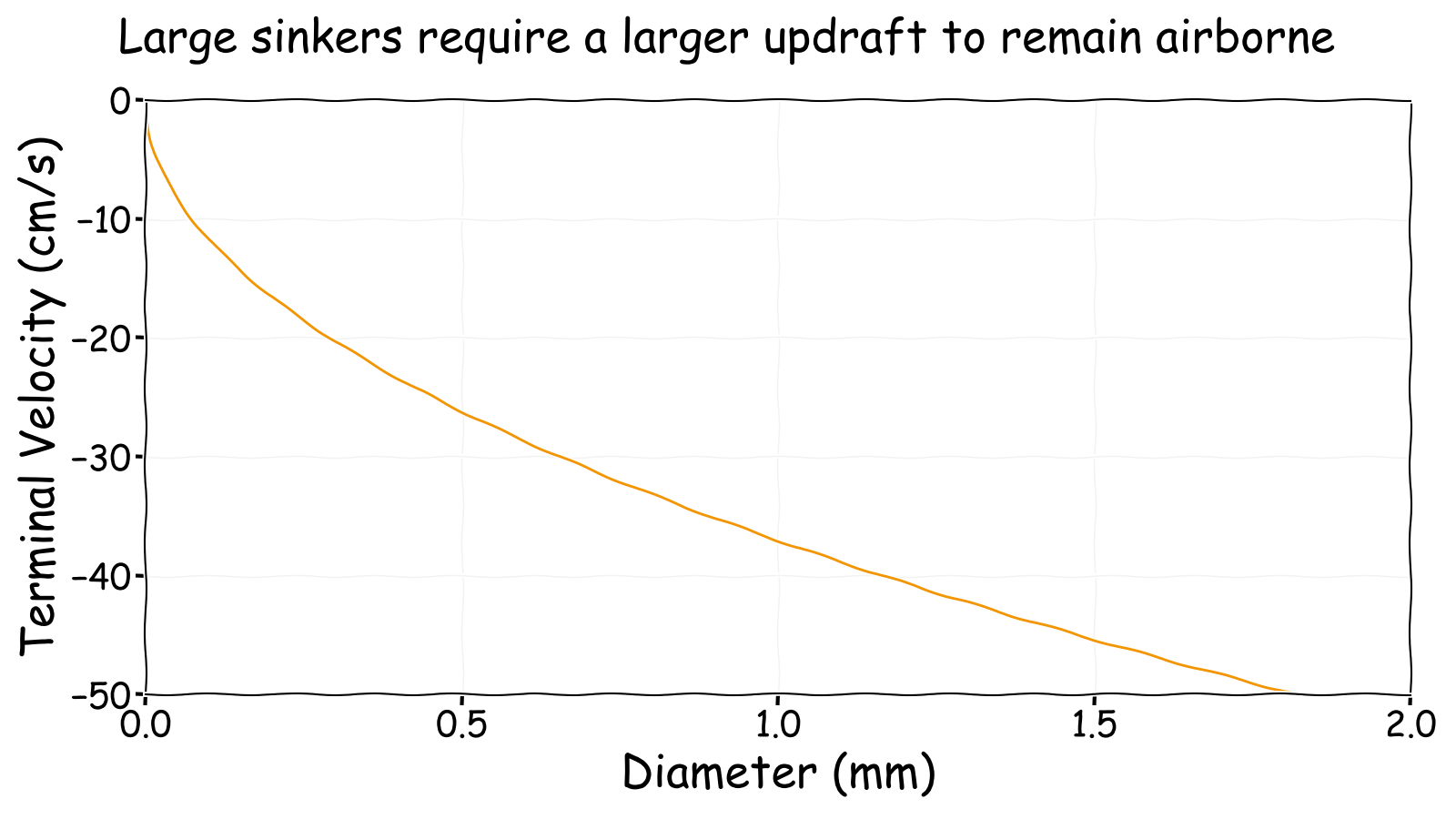
This is only a crude model but it suggests that larger sinker aeroplankton would require vertical wind speeds at least ten times greater to remain permanently airborne without flapping their wings. This probably isn’t a widespread occurrence but in specific locations such as near mountains, dark areas that warm to create thermal updrafts or the sub-stellar point on a tidally locked planet it may be plausible for extended periods. Small “sinkers” could soar to high altitudes in these regions and then glide slowly down to begin the cycle anew.
Larger sinkers are somewhat viable but they may not be able to remain permanently airborne outside of specific areas without flapping wings.
Electrostatics
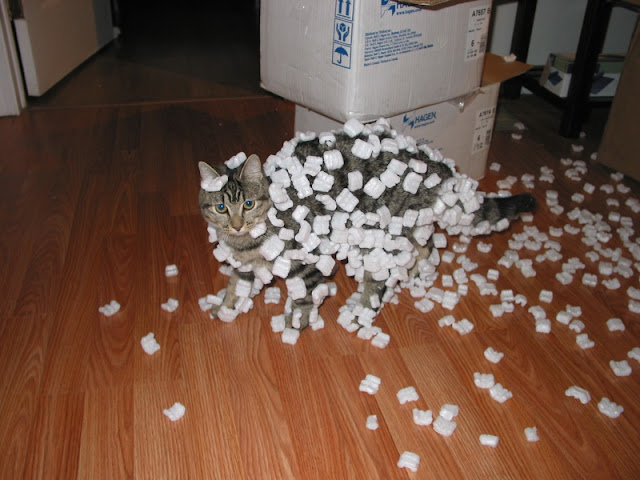
The final mechanism that might allow aeroplankton to passively remain airborne for long periods of time is electrostatic repulsion. This is the repulsive force between two objects with the same electric charge. In contrast, when objects (such as fur and polystyrene) become oppositely charged then the force becomes attractive.
Ballooning spiders are actually known to use electrostatic repulsion to fly. Due to the global atmospheric electric current the Earth’s surface is negatively charged and an electric field of around 120 V/m exists at ground level in fair weather conditions. Spiders produce threads of silk which become negatively charged and thus produce a repulsive force between the spider and ground. The result of this is that spider is lofted into the air.
This is a thinly researched area, so I won’t model this but will just state that spiders with a mass of around 0.2 mg have been observed at altitudes of 4 km. A single strand of silk perhaps 20 cm long is used to achieve this though multiple thread configurations are also possible. In fact, the process by which silk becomes charged when it is spun may have evolved to keep multiple strands apart, though much less charge is required to achieve this than to fly.
Hypothetical silica coated aeroplankton could also become charged through collisions with other sand particles. This process is believed to cause charge separation and subsequent lightning in volcanic plumes, sandstorms and even the dust blown up by helicopters in the desert.
Of course, these techniques probably wouldn’t allow permanent flight as eventually the charge would dissipate and the electrostatic repulsion would fade. It is however possible that additional positive charge could be accumulated in the upper atmosphere where the interaction with the solar wind produces charged particles. A photosynthetic or chemosynthetic process could perhaps generate energy that could be used to separate charges too.
Interestingly, a large electrostatic “glider” has been proposed for use in space for inspecting asteroids due to the presence of charged dust particles. Perhaps something like this could produce a large electrostatic gasbag on another planet?
Electrostatic repulsion could perhaps be used to help aeroplankton float via charged silk strands or silica shells.
Conclusions
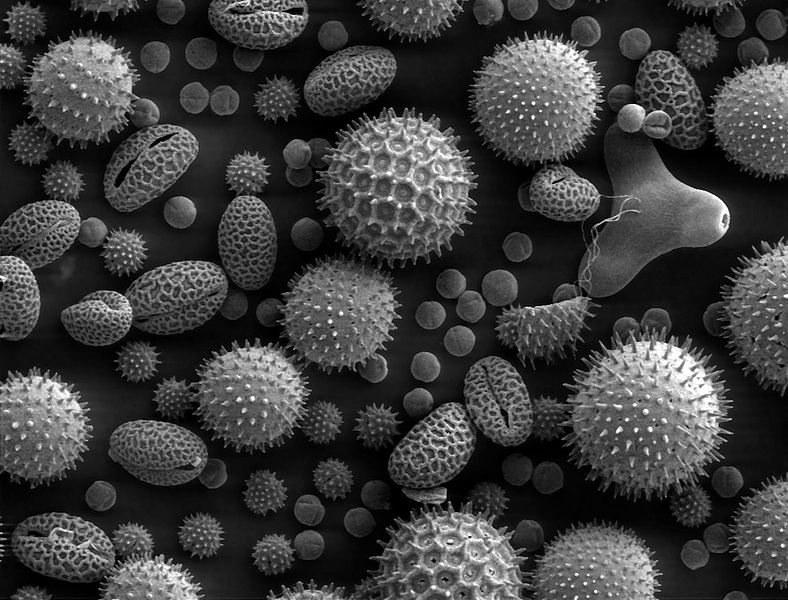
In summary, it does at least appear to be possible for aeroplankton of various sizes to exist and use various approaches (e.g. buoyancy, updrafts and electrostatic repulsion) to remain in the air for extended periods of time. They can be split into two categories, floaters and sinkers, depending on whether they are permanently lighter-than-air or have to cycle up and down through the atmosphere.
I have not yet investigated other aspects of aeroplankton such as how they acquire energy, carbon, water and nutrients. This is certainly a potential problem but as they same issues face the larger gasbags previously discussed I will leave that for the next post. That will cover these and other anatomical questions that related to life in a permanent aerial ecosystem.
Overall, the presence of some level of aeroplankton is easy to justify under a range of planetary conditions as it has already occurred on Earth. However, this seems to just be passing through rather than permanently dwelling the clouds. It may be possible to plausibly justify a rich aerial ecosystem on another planet where aeroplankton act as the base of the food chain and this will be the topic of the final post in this series.
Acknowledgements
I would like to thank Reddit user 123Thundernugget for letting me use their recently posted artwork of aeroplankton. It nicely illustrates the range of possibilities that are covered with broad analysis I described above.